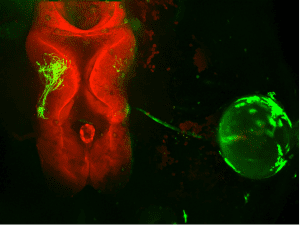
It has long been appreciated that sensory experience helps to refine the connectivity of the brain during development. In 1949, Canadian psychologist Donald Hebb proposed that when different brain cells were consistently active at the same time as one another and acted in synchrony, the connections they formed would be strengthened as a result of their cooperation. This so-called “Hebb rule” for circuit remodeling is sometimes restated as “cells that fire together, wire together.”, and helps explain how the wiring of the brain could be fine-tuned in response to sensory input. Fast forward 70 years and many of the artificial neural networks we rely on today to make accurate predictions from large datasets rely on digital implementations of various learning rules, including variants of Hebb’s rule, that underlie their ability to learn associations.
A quarter century after Hebb, the brilliant molecular biologist turned neuroscientist, Gunther Stent, proposed that there should also be a kind of reverse Hebb rule for nonconformist neurons that fire out of synchrony with their neighbors. Such neuronal “social distancing”, Stent argued, should result in modifications opposite to that described by Hebb whereby cells that “fire out of synch, lose their link” with their partners. In principle, Stent’s addition to Hebb’s rule should be far more efficient at refining networks, both in the brain and digitally, than Hebb’s rule alone.
Thanks to a tiny tadpole, and a very big laser, researchers in the Montreal Neurological Institute-Hospital at McGill University have at last been able to demonstrate the existence of this alternative learning rule, which they named “Stentian plasticity”, in real time in the brain. McGill University medical student Tasnia Rahman, working in the laboratory of Dr. Ed Ruthazer, took advantage of the small transparent brains of tadpoles, which undergo the same kinds of developmental fine-tuning as human brains, and genetically expressed green fluorescent protein from bioluminescent jellyfish in single cells in their eyes. The retinal cells extended long projections called axons into the brains of the tadpoles which could then be observed using a powerful 3-dimensional microscopy technique that sends intense pulses of laser light deep into the tadpole brains, causing the fluorescent retinal cells to glow and become visible in the intact animal. Rahman then presented visual stimuli to the eyes of the tadpoles that caused the labeled axon to “fire out of sync” with its neighbors, recreating the conditions described by Stent.
The experimenters were surprised to discover that when the renegade neuron remained silent while its neighbors were cooperatively firing with each other, it would actually grow faster and further than the cells that were firing together. Interestingly, when the sole axon fired by itself this phenomenon was not observed. So this meant that there was a signal generated by the quiescent cell’s active neighbors that effectively told the nonconforming axon to “get out of here” by growing faster. This makes sense as a means to facilitate the sorting of an initially chaotic set of inputs into more organized neural representations by expelling inputs that don’t belong based on their mismatched activity patterns. The group is now working to understand how much more efficient than a simple Hebb rule is the addition of this Stentian plasticity rule for circuit refinement and whether this finding might help promote better learning in artificial neural networks as well.
Original Research Article:
Rahman et al.,(2020) Stentian structural plasticity in the developing visual system. Proc Nat Acad Sci USA. https://doi.org/10.1073/pnas.2001107117