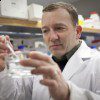
Synaptic plasticity, or the ability of neurons to form, strengthen, or weaken connections with each other, has long been studied as the basis for learning and memory. While the cellular processes and biological mechanisms involved are complex, much progress has been made at the Brain Research Centre and other research institutes around the world towards understanding this dynamic brain process.
What is now well-established is that external stimulation causes synapses to strengthen or weaken their connections, which in turn affects the growth and development of neurons. This is learning at the cellular level, and Kurt Haas, a member of the Brain Research Centre, runs one of the best labs in the world for imaging individual neurons in 4D to study this process.
In recent years, attention has begun to shift towards the concept of metaplasticity, or the plasticity of synaptic plasticity—that is, how external stimuli and past history of synaptic activity together determine synaptic responses.
“As synapses change in response to external stimuli, scientists have hypothesized that something must be at play to stop synapses from going to the extremes over time—that is, something must be stopping these feed-forwarded processes promoting strong synapses to become stronger, or weaker ones to become weaker,” says Dr. Haas. “Without such controls, strong synapses would eventually reach a ceiling in which they are maximally strong and can no longer respond to further stimuli and theoretically no more learning could occur.”
That “something” is the process of metaplasticity. Its mechanisms keep synaptic strengths and responses to within a physiologically dynamic range that still allow for an increase or decrease in connection strength in response to future input.
If the concept of metaplasticity is a challenging one to understand, then devising a scientific method to explore this phenomenon is ever more so. However, three years ago, Dr. Haas did exactly that: he established a powerful new model system to study plasticity and metaplasticity mechanisms in isolation, a requirement to disentangle the molecular mechanisms of each. In doing so, he provided some of the first evidence that visual stimulation can induce coordinated changes to developing brain circuits.
Now, he’s made even bigger strides by identifying a molecular cascade and a critical molecular switch—MEF2, a transcription factor—that controls this coordinated response. He and his team also discovered that MEF2 is regulated by a pathway involving caspases, which are well-known proteins that mediate apoptosis (a normal biological function of programmed cell death). This represents an important newly recognized non-death role for caspases in the brain.
The results of this study were published in the September 28 edition of the journal Cell.
“We now have potential new targets to explore for involvement in neurodevelopmental disorders and for development of new drugs or therapies for their treatment,” says Dr. Haas, who is also an Associate Professor in the UBC Department of Cellular & Physiological Sciences. “However, we first need to figure out the cellular and molecular mechanism by which MEF2 is acting to induce these metaplastic changes, and to find the downstream gene targets that directly regulate plasticity thresholds at the synapse.”
This study was funded by grants from the Canadian Institutes of Health Research, the Michael Smith Foundation for Health Research, the Tula Foundation, and the EJLB Foundation.
Source of text: UBC Brain Research Center
Photo credit: Martin Dee, UBC Public Affairs.